From rare multimessenger events to large-scale statistical methods, gravitational-wave observations are paving the way toward a deeper understanding of the cosmos.
Professor Archisman Ghosh is an Assistant Professor in the Department of Physics and Astronomy at Ghent University. He specialises in gravitational-wave (GW) data analysis, with a research focus on uncovering insights into fundamental physics and cosmology from GW observations. His work spans current detectors – such as the LIGO-Virgo-KAGRA (LVK) network – as well as next-generation observatories including the Einstein Telescope and the Cosmic Explorer. In recent years, Professor Ghosh and his research group have been exploring how GW observations can help measure the Hubble constant – the rate at which the universe is expanding today – offering an exciting new window into our evolving cosmos.
What is the question that you are trying to answer?
We still do not know how fast our Universe is expanding! Over the past decade, a striking discrepancy has emerged between different measurements of the universe’s expansion rate made using local probes and inferred from the early universe. This puzzle – now famously known as the Hubble tension – may point to unknown systematic errors in cutting-edge measurements or suggest that our current cosmological model is incomplete.
Gravitational waves, directly detected for the first time in 2015, offer a revolutionary and independent method for probing the cosmos. They provide a fresh and complementary way of measuring the Hubble constant and other fundamental parameters of cosmology. Unlike conventional approaches, GW-based measurements are free from the assumptions underpinning the existing methods in tension. This opens up a powerful opportunity: GWs could help resolve the Hubble tension and illuminate new physics underlying our evolving universe (Fig. 1).

How are gravitational waves able to help?
Gravitational-wave observations are truly unique. Unlike most electromagnetic (EM) observations, which are typically sensitive only to the overall intensity of light averaged over many phase cycles, GW detectors capture both the amplitude and phase of the signal. This provides a much richer source of information.
In the case of merging compact binaries – such as black holes or neutron stars – the way the signal’s phase evolves encodes crucial details about the system, including the masses of the objects involved. Since the amplitude of a gravitational wave diminishes in a well-understood way with distance (specifically, as one over the square of the distance), it becomes possible to directly measure how far away the source is. This makes these systems powerful ‘standard distance indicators,’ or as we call them in this context, standard sirens.¹
However, to determine cosmological parameters such as the Hubble constant, measuring distance is not enough. One also needs to know the redshift – how much the signal’s frequency has been stretched due to the expansion of the universe. Redshift is difficult, and often impossible, to determine from GW data alone. This is where complementary information becomes essential.
Currently, there are three main approaches to obtaining redshift:
- Bright standard sirens: When a GW source has a clearly identified EM counterpart, such as a flash of light from a neutron star merger, the redshift can be measured from that EM signal.
- Spectral sirens: By examining how the distribution of observed source masses appears to shift due to redshift, one can statistically infer redshift information.
- Dark standard sirens: If no EM counterpart is observed, but the region of sky where the GW originated is known, a galaxy catalogue can be used to match potential host galaxies and their redshifts.
There are also other innovative methods for estimating redshift, although these are more likely to be applicable with future, more sensitive GW detectors.
What measurements have we made so far?
The binary neutron star merger GW170817, detected by the LIGO-Virgo network, marked a breakthrough moment. It was the first GW event with a bright EM counterpart, observed across multiple wavelengths. This allowed astronomers to quickly pinpoint its host galaxy and measure its redshift. With this information, we obtained the first-ever gravitational-wave-based measurement of the Hubble constant, albeit with a relatively broad uncertainty of about 14%.² If we were to observe around 100 similar events, the precision would come down to around 1.4%. However, such multimessenger events remain extremely rare; to date, GW170817 is the only confirmed case.
In the meantime, attention has turned to spectral and dark standard sirens. Most of the gravitational-wave events detected so far do not have electromagnetic counterparts. From the first three observing runs of the LIGO-Virgo-KAGRA collaboration, we have collected about 90 such events. In the current fourth observing run, we already have more than 200 candidate events, none with confirmed EM counterparts.
Despite the lack of direct redshift information, these events are far from useless. By applying spectral and dark siren methods, we can statistically extract cosmological information from the growing population of detections.3,4,5,6 While each individual event provides only a small piece of the puzzle, together they gradually build up a clearer picture, offering a powerful way to contribute to the measurement of the Hubble constant, even without light.
What research is your group specifically focusing on?
One of the main challenges in using dark sirens is the severe incompleteness of galaxy catalogues. Most existing catalogues provide reliable redshift information only up to z≈0.25 over the majority of the sky. However, GW sources are already being detected from far greater distances, and this range will only continue to increase as the sensitivity of the LVK detector network improves. To make full use of these more distant sources as dark standard sirens, we need to turn to alternative redshift tracers.
My group is actively exploring the use of galaxy clusters and bright galaxies as such alternatives. These objects are visible out to significantly higher redshifts and, although they only approximately trace the underlying matter distribution associated with gravitational-wave source localisations, this level of accuracy is often sufficient. This is because the distance measurements from GWs themselves come with relatively large uncertainties, typically around 10%.
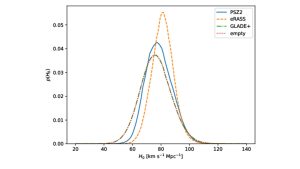
In recent work, we have shown that galaxy cluster catalogues, constructed using the Sunyaev-Zel’dovich effect on the cosmic microwave background (CMB), or using X-ray surveys, can improve the precision of Hubble constant measurements by 10% and 40%, respectively (Fig. 2).⁷ Furthermore, using brightest cluster galaxies could lead to improvements of up to 80% in the most optimistic scenarios (Fig. 3).⁸ These are promising results from initial studies, and we are currently developing and rigorously testing these methods to establish them as robust and viable data analysis pipelines.

Such approaches will not only be helpful – they will be essential as the LVK network grows more sensitive and as next-generation observatories like the Einstein Telescope (ET) and Cosmic Explorer (CE) come online.
Do you think that gravitational waves will resolve the Hubble tension in the coming years?
I am confident that gravitational waves will provide valuable and independent information that will help clarify the Hubble tension. Importantly, we do not need to reach 1% precision for GW-based measurements to be impactful. Even with uncertainties at the level of a few percent, we may already begin to see agreement with one side of the current tension, or neither.
That said, predicting exactly when this will happen is challenging. The timeline depends on several factors, including the number of detections and the nature of the sources. However, we can accelerate progress by expanding the number of GW events that can be used for cosmological inference, particularly through methods like using alternative redshift tracers.
One critical task is to identify and control all potential sources of systematic error, ensuring they never dominate over the statistical uncertainties. This is no small feat, but the community is already deeply engaged in this effort, and we are actively contributing to it.
Gravitational-wave measurements are fundamentally independent of traditional methods. The sources of systematic error in GW observations are entirely different from those affecting existing electromagnetic measurements. Moreover, GWs allow for direct distance measurements at any redshift, without the need for a local or high-redshift anchor. This means we can go beyond measuring just the local expansion rate (the Hubble constant) and begin probing directly the time-dependent Hubble parameter – the full expansion history of the Universe.

Looking ahead, future detectors will take us even further. They will enable us to explore the cosmic ‘dark ages’ – an era from which no significant electromagnetic signals reach us. Gravitational waves are the only messengers which will directly probe this otherwise invisible chapter of cosmic history.
References
- B. F. Schutz, Nature 323 (1986) 310–311.
- B. P. Abbott et al., Nature 551 (2017), no. 7678 85–88.
- B. P. Abbott et al., Astrophys. J. 909 (2021), no. 2 218.
- R. Abbott et al., Astrophys. J. 949 (2023), no. 2 76.
- R. Gray et al., JCAP 12 (2023) 023.
- S. Mastrogiovanni et. al., Phys. Rev. D 108 (2023), no. 4 042002.
- F. Beirnaert et al., arXiv:2505.14077 [astro-ph.CO].
- K. Naveed et al., arXiv:2505.11268 [astro-ph.CO].
Please note, this article will also appear in the 22nd edition of our quarterly publication.